How to trap antimatter and matter together
The APEX Collaboration, centered around the APEX-PAX-EPOS working group in Garching, aims to research plasmas consisting of electrons and positrons.
For this purpose, the team has now built at the Max Planck Institute for Plasma Physics (IPP) a state-of-the-art experiment that is unique in the world. Its most important component: a high-temperature superconducting magnetic ring that levitates freely in vacuum and thereby can capture charged particles, positive and negative. It will enable experiments on matter-antimatter plasmas that have never been able to be done before.

Scientist Alexander Card experienced a most beautiful moment this past summer, when a shiny gold ring with a diameter of 15 centimetres detached itself from a platform. The platform had previously lifted the ring into the centre of a vacuum chamber at a speed of just a few millimetres per second. Then for more than a minute, the ring appeared to fight for its freedom. Above, a growing magnetic field pulled the ring upwards. From below, however, the platform held on stubbornly, due to its own induced magnetic fields. The ring trembled, initially lifting off more on one side than the other as it tested the levitation control system, before finally floating completely freely and stably – a state that subsequently lasted for three and a half hours.
PhD student Alexander Card (Technical University Munich, IPP) has spent four years working towards this moment, in collaboration with colleagues from IPP, THEVA (superconducting tape manufacturer in Ismaning), and Prof. Matthew Stoneking from Lawrence University in the United States. As part of his doctoral thesis, he has solved a series of novel experimental challenges, in order to make this so-called levitated dipole trap a reality. "There have been much larger levitated dipole experiments at the University of Tokyo and at MIT, the Massachusetts Institute of Technology in the USA, but we have engineered our setup to be uniquely suited to trap pair plasmas of matter and antimatter."
Plasmas with symmetrical mass distribution
Plasma is what physicists call the fourth state of matter. If, for example, hydrogen gas is heated above a certain temperature, a plasma is created: the hydrogen atoms then split into charged particles – i.e. electrons and ions (in the case of hydrogen, these are protons). In such a plasma, the masses are distributed very asymmetrically – a proton is 1836 times heavier than an electron. The plasmas produced in the nuclear fusion experiments at ASDEX Upgrade and Wendelstein 7-X are thus extremely asymmetrical in this way.
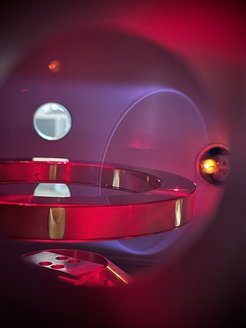
"We would like to study ideal plasmas in which the masses are symmetrically distributed," says working group leader Dr Eve Stenson, explaining her team's field of research. Compared to most investigations at IPP, this work is not as directly focused on future nuclear fusion power plants. "We want to get to the bottom of fundamental physical phenomena, though there is always the chance we might discover something useful for understanding fusion plasmas as well." The name of the collaboration describes the ultimate goal: APEX (A Positron-Electron Experiment), and thus the levitated dipole trap is dubbed APEX-LD.
The broader APEX Collaboration also includes the PAX (Positron Accumulation eXperiment) and EPOS (Electrons and Positrons in an Optimized Stellarator) experiments at IPP, in addition to related efforts with collaborators at universities in Germany and internationally. The collaboration has received support from many third-party funding agencies (including the ERC, DFG, Helmholtz Society, U.S. Department of Energy, Humboldt Association).
Positrons, which are positively charged, are the antiparticles of electrons. Except for the opposite electric charge (and related quantities, such as magnetic moment), the two have exactly the same properties. For reasons that are as yet unknown, though, antimatter is far less abundant in our universe than matter. "We hear about matter-antimatter plasmas mainly from science fiction," says Dr Stenson. "However, they actually exist in astrophysical environments – for example, near quasars, the bright centres of galaxies." In addition, these pair plasmas are thought to be found in the accretion disks of young galaxies, the rotating disks that transport matter to the centre of these star systems. In an early phase of our universe, such plasmas probably formed the predominant state of matter.
It is widely known that when electrons and positrons combine, the antiparticles annihilate each other within a very short time, their mass converted into energy in the form of gamma rays. With a suitable trap and low densities of the two species, however, the immediate annihilation of all particles can be delayed and a plasma of both types of particles can be maintained and controlled in the laboratory.
Glowing electron paths
This is precisely the purpose of the levitating dipole trap at IPP. Electrons and positrons introduced into the vacuum chamber are constrained by the Lorentz force to follow circular and helical paths around the magnetic field lines that encircle the floating superconducting ring. Because the two species' electrical charges are opposite, they also drift in opposite directions around the ring – i.e. either clockwise or counterclockwise (when viewed from above).
The plasma in the vacuum vessel will have a relatively low density, so that the antiparticles can only meet and annihilate each other comparatively rarely. Nevertheless, the collaboration expects about 100 million such annihilations per second in the experiment. Members of the team at IPP have been developing diagnostics to gain insights into the plasma properties by analysing the gamma radiation released.
At least with electrons, doctoral student Alexander Card can already demonstrate the capabilities of his trap. An electron emitter with adjustable current and voltage is part of the apparatus. You can even follow the spiral path of the electrons with the naked eye as blue glowing traces if you look through one of the portholes in the vacuum chamber. If you introduce some helium into the vacuum chamber, the electrons excite the gas to glow.
"In order to be able to experiment with pair plasmas, we will move the apparatus next year to the research neutron source FRM II, which also produces positrons," explains Dr. Stenson. FRM II is located in the immediate vicinity of the IPP on the Garching research campus, and it hosts theNEutron-induced POsitron source MUniCh (NEPOMUC), led by TUM Prof. Christoph Hugenschmidt, which is the most intense "cold" (i.e., low-temperature) positron source worldwide. FRM II is currently undergoing a multi-year maintenance phase.
How the levitating dipole trap works
The levitating dipole trap is ready for operation. Alexander Card has even programmed and perfected the experiment so that the floating magnetic ring moves into position at the touch of a button. This hides the expert knowledge that needed to be developed to coordinate the precise interaction of three electromagnetic coils.
The C coil (for "charging"; diameter 24 centimetres) is mounted on the floor of the vacuum chamber. Its 410 turns are wound from high-temperature superconducting material. From a maximum temperature of minus 225 degrees Celsius, current flows in it without loss, i.e. without electrical resistance. To achieve this, the magnet is cooled by a coldhead (a system that operates a refrigeration system based on high-pressure helium gas). Its function: It charges the F coil – the gold ring that will later float – by electromagnetic induction.
The F coil (for "floating"), also consists of high-temperature superconducting windings – there are 132 of them. Induction causes a current to flow loss-free in the ring (which has also been cooled to minus 250 degrees Celsius), and this in turn generates a magnetic field in dipole form. For this charging, the F coil lies inside the C coil at the beginning of the experiment routine, before a lifting platform moves it into the centre of the 162-litre vacuum vessel.
There the L coil (for "lifting") comes into play; it is located outside the vacuum vessel and consists of ordinary copper conductor. It pulls the gold ring upwards against gravity and then holds it precisely in its suspended position. Three lasers directed at the ring from above, through windows in the vacuum chamber, ensure that the deviation is limited to a maximum of 0.02 millimetres. Detectors can measure tiny deviations from the reflected laser beam and report them to the experiment's control system. This then varies the field of the L coil accordingly so that the position is always maintained.
Over time, the temperature in the levitating ring rises so that a resistance builds up in the solder joints between the ends of the high-temperature superconductor. The current in the F coil gradually decays, and to keep it in place, the current in the L coil must increase steadily. When it exceeds a certain threshold value (above which overheating of the L coil might become a concern), the experiment is concluded – in the tests so far, this was after three and a half hours. The current in the L coil is lowered while the lifting platform picks up the ring magnet again. The charging cycle starts all over again.
Alex Card captured the entire procedure on video, documenting the sophistication of the experiment. "We are very excited to reach this milestone, having overcome many technical challenges, as well as other obstacles," says working group leader Stenson. The project was significantly affected first by the pandemic, then by the subsequent supply chain issues; it also had to reckon with many unknowns in the process of developing new technologies on the way to operation. "It was little more than a year ago that we didn't yet know how well induction would work, how quickly the induced current would decay, what a quench of the F coil would look like, how long or how stably we would be able to levitate. Now we are looking forward to the start of the scientific experiments."
Frank Fleschner